| Faults and fractures
James S. Aber, Professor Emeritus
Emporia State University, Kansas
|
Fractures and joints
| Pegmatite vein (left) fills a fracture in the Svaneke granite. Notice the large quartz and feldspar crystals of the pegmatite. Scale pole marked in 20-cm stripes. Nexø arkosic sandstone (right), basal Cambrian strata. Well-defined joints are utilized to quarry the rock for building stone. Island of Bornholm, Denmark (Bruun-Petersen 1977).
| |
| Basaltic dike and granite (left). The basalt intruded a fracture in the granite. Close-up view (right) of dike/granite contact. Rapid cooling of magma at the dike margin led to quick crystallization and a fine-grained (aphanitic) texture. Island of Bornholm, Denmark.
| 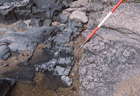 |
| Deep cleft on the sea cliff at Johs Kapel. Overview (left) and close-up shot (right). The cleft has formed by erosion and removal of mafic dike rock from between walls of granite. Vertical sea cliff marks one side of the eroded dike. Island of Bornholm, Denmark (Jørgart 1977).
| 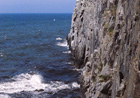 |
| Two vertical joints (> <) meet at a near right angle in the Cottonwood Limestone (left). A single, vertical joint plane in the Fort Riley Limestone (right). Lower Permian, Flint Hills of Kansas. Scale pole marked in feet. Such joints are ubiquitous in bedrock of the U.S. mid-continent region.
| 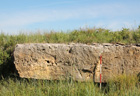 |
| Fractured and rotated flint nodules within heavily brecciated Cretaceous chalk, a result of glaciotectonic deformation. Weybourne, United Kingdom. Pocket knife for scale.
| 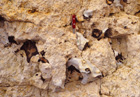 |
| Fractured cobbles within a drumlin. Fracturing was caused by vertical loading of an overriding ice sheet. Silva compass for scale. Dollard, southern Saskatchewan, Canada (Kupsch 1955).
| 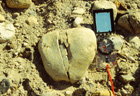 |
| Quartz veins fill gash fractures that formed from tension. Pocket knife for scale. Dingle Bay, Ireland.
|

Landslides in Alberta, Canada
| Police Point landslide (left), Cypress Hills, southeastern Alberta. This landslide developed on a north-facing slope in the 1960s. Undisturbed upland surface to left and slumped fault blocks to right. Lower portion of the landslide (right) displays more extensive disruption of fault blocks.
| 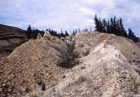 |
| Frank landslide (left) in the Rocky Mountain front range, southwestern Alberta. A large mass of rock failed suddenly from the mountain side on April 29, 1903. The resulting landslide buried the town of Frank at the valley bottom and killed 70 people. Landslide debris flowed up the opposite side of the valley (right) and deposited mounds of jagged boulders across the scene.
| 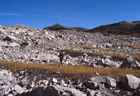 |
| Tilted ledges of sandstone bedrock are exposed in an old (Holocene) landslide in Medicine Lodge Coulee, Cypress Hills, southeastern Alberta. Silva compass indicates moderate angle of dip.
|

Faults and fault zones
| Common types of faults. Normal fault (left) in which the hanging wall has moved down. Note slight drag folding along the fault. Thrust fault (right) in which the hanging wall has moved up. Scale pole marked in feet; faults shown by red lines. Lower Permian strata, Flint Hills, Kansas.
| 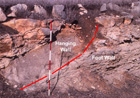 |
| Fault zones in gneiss bedrock of the Hudson Highlands, eastern New York. Discrete fault (left) with well-defined drag folds. Pegmatite (right) marks a fault, again with drag folds. Sunglasses for scale.
| 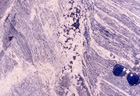 |
| Front of the Santa Elena fault, Big Bend National Park, Texas. Left: lower Cretaceous limestone forms the cliff on the uplifted side of the fault (background), and upper Cretaceous chalk is seen on the downthrown side at the base of the cliff. Close-up view of the Santa Elena fault (right), marked here by numerous veins of large calcite crystals. Such secondary mineral deposits are typical of major faults.
| 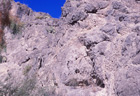 |
| Crest of anticline in the upper Cretaceous Whitemud Formation in the Cactus Hills, a set of large ice-shoved ridges in southern Saskatchewan, Canada. The anticline was produced by ice pushing and upthrusting of displaced bedrock. The anticline is disrupted by small faults (see below). |
| Normal faults on the crest of anticline (left). These faults were created by glacial loading after ice advance overrode and truncated the fold crest. Thrust fault (right) on the upice flank of the Cactus Hills anticline. Lignite seam (black) is repeated. Note how lignite is smeared along the fault for a distance of several meters. Scale pole marked in feet.
| 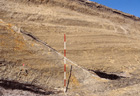 |
| Glaciotectonic faults from Denmark. Left: small thrust in poorly consolidated Jurassic sandstone pushed over glacial gravel. Scale pole marked in 20-cm stripes. Right: opposed thrust faults create a downdropped block (graben) in the Fur Formation (diatomaceous clay and volcanic ash layers). Note smaller thrust and kink folds at upper left. Field of view ~3 m (10 feet) across.
|
|
Overthrust faulting
The major structures underlying mountain belts and ice-shoved hills are long, low-angle thrust faults called overthrusts. A major overthrust may represent a décollement, which is a structural break separating complexly deformed rocks above from undeformed basement rocks below. The displaced rock mass above the décollement is said to be allochthonous, in contrast to the unmoved autochthonous basement rocks below the décollement. The allochthonous rocks have often been transported laterally for great distances (10s to 100s km). Décollements are known to underlie complexly folded strata in many mountain belts—Swiss Juras and Alps, Appalachians, Ouachitas, Canadian Rockies, and Himalayas. Décollements are associated also with large ice-shoved ridges in many formerly glaciated regions.
How such large rock masses could be pushed or slid laterally
for great distances was long a puzzle to geologists. The physical
conditions necessary for overthrusting of large bedrock blocks
were elegantly explained by Hubbert and Rubey (1959), who
analyzed subsurface pressure conditions. The lithostatic pressure developed in deeply buried rocks is comprised of two components.
- Intergranular pressure – pressure transmitted directly from grain to grain within the rock body.
- Hydrostatic pressure – pressure transmitted indirectly by fluid filling pore spaces or fractures between grains.
Below the water table, all rocks are completely saturated with fluid of some kind—water, oil, brine, or gas. Thus, subsurface pressure conditions may be represented as:
- P(lithostatic) = P(intergranular) + P(hydrostatic)
In most cases, the lithostatic P is borne mainly by intergranular P, and hydrostatic P is much less than lithostatic P. However, in some situations, hydrostatic P may approach or equal lithostatic P, in which case the overburdern mass of rock essentially floats on a cushion of high-pressure fluid. Hubbert and Rubey (1959) demonstrated that, as hydrostatic P reaches lithostatic P, the shear stress required to move the overburden approaches zero. This condition is nicely illustrated by the game of "air hockey," where the puck slides effortlessly over a film of high-pressure air coming through tiny holes in the playing surface.
The necessary fluid pressure may be developed in the crust
when porous, mechanically weak strata of low permeability, such
as claystone, lignite, chalk, or salt, are subjected to rapid
loading. The grain-to-grain structure of the rock may collapse,
and weight of the overburden is then borne by a fluid cushion
trapped within the rock. This raises the fluid pressure of the
rock, expells some fluid from the rock, and compacts the rock.
Rapid loading may compact incompetent strata raising the
hydrostatic P in various settings—thick buildup of orogenic sediments (flysch), prograding delta, accumulating volcanic deposits, advancing ice sheet, or pushing of a thrust block onto previously undisturbed strata. The high fluid pressure sufficient to facilitate overthrusting may develop whenever the rate of loading for whatever reason is greater than the rate of fluid escape from compacted, weak strata.
Once a cushion of high-pressure fluid is created, lateral movement of the overlying rock may be accomplished by pushing, as when plates collide or a glacier advances, or simply by gravity sliding down a gentle gradient. In any case, overthrusting will continue only so long as high fluid pressure is maintained within the décollement.
| Simpson Pass thrust fault, Canadian Rocky Mountains front range, western Alberta. Cambrian strata (left, dark) are thrust over Ordovician rocks (right, light). This is a classic example of a large montane thrust in which older strata come to rest on top of younger rocks. |
| Major thrust fault in the Culebra Range, Sangre de Cristo Mountains, southern Colorado. Overview (left) and close-up shot (right). Reddish colored Proterozoic crystalline rocks (*) are thrust up and over Pennsylvanian sandstone and shale. The fault zone is broken and jumbled.
| 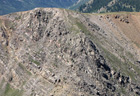 |
| Overthrust of brown Eastend Formation onto white/gray Whitemud Formation (left). In the normal stratigraphic sequence, Eastend underlies the Whitemud. Detail (right) displays overturned drag folding in the Eastend thrust over the Whitemud. Scale pole marked in feet. The Dirt Hills are a huge ice-shoved ridge complex built of displaced upper Cretaceous strata, southern Saskatchewan, Canada.
|
|
References
- Bruun-Petersen, J. 1977. Nedre Kambrium. In, Geologi på Bornholm, p. 33-39. Varv, Ekskursionsfører Nr. 1.
- Hubbert, M.K. and Rubey, W.W. 1959. Role of fluid pressure in mechanics of overthrust faulting. Geological Society America, Bulletin 70, p. 115-166.
- Jørgart, T. 1977. Prækambrium. In, Geologi på Bornholm, p. 8-32. Varv, Ekskursionsfører Nr. 1.
- Kupsch, W.O. 1955. Drumlins with jointed boulders near Dollard, Saskatchewan. Geological Society America, Bulletin 66, p. 327-338.

Return to Table of Contents.
Notice: Advanced tectonics is presented for the use and benefit of students enrolled at Emporia State University. Others are welcome to view the course webpages. Any other use of text, imagery or curriculum materials is prohibited without permission. All text and imagery © J.S. Aber (2021).