Lobate pattern of glaciation
Chamberlin (1882) described the terminal (Late Wisconsin) moraine thusly:
It constitutes a broad, irregular range of confusedly heaped drift
rather than a simple continuous ridge or group of definite parallel ridges
... Genetically considered, it embraces two or more such ranges, which
sometimes coalesce into a common massive belt, and sometimes separate
... (p. 310).
He continued: The superficial aspect of the formation is that of an
irregular, intricate series of drift ridges and hills of rapidly, but often
very graceful, undulating contour, consisting of rounded domes, conical
peaks, winding and occasionally geniculated ridges, short, sharp spurs,
mounds, knolls, and hummocks, promiscuously arranged, accompanied by
corresponding depressions, that are sometimes even more striking in
character.
On moraine loops: It is disposed in a series of great loops which give
to the whole a peculiar arcuate expression. A remarkable peculiarity is
the extraordinary fact that the lateral portions of these great loops do
not simply join, forming re-entrant angles, but coalesce and are prolonged
sometimes a hundred miles (160 km) or more between the lobate areas
embraced by the loops, forming a peculiar morainic type, to which the term
intermediate or interlobate moraines will be applied. (p. 313)
Chamberlin recognized twelve such great loops (lobes) encompassing broad
valleys in the northern United States--see Table 8-1.
Table 8-1. Twelve large ice lobes of the southern glacial margin in the United States, as originally mapped by Chamberlin (1882).
Eastern United States | Central United States |
Champlain-Hudson lobe, N.Y. |
Lake Michigan lobe, IL/WI. |
Finger Lakes lobe, N.Y. | Green Bay lobe, Wisconsin. |
Grand River lobe, Ohio. | Chippewa lobe, N. Wisconsin. |
Scioto lobe, Ohio. | Superior lobe, MN/Wisconsin. |
Maumee lobe, Ohio/Indiana. | Des Moines lobe, MN/Iowa. |
Saginaw lobe, Michigan. | James valley lobe, N/S Dakotas. |
On-campus ESU students, see geologic maps of the northern Great Plains and Great Lakes regions on display in Science Hall (maps #24 & #25, next to room 128). These maps depict lobate patterns of glaciation.
| Hudson River, New York: NASA space-shuttle photo, STS58-81-038,
70 mm format. Low-oblique view toward northwest over Long Island, New York
City, Hudson River, and Catskill Mts. Reddish-brown color shows autumn foliage
of forest in Catskills, October 1993. During the late Wisconsin glaciation, an
ice lobe extended southward along the Hudson valley and reached a maximum
position marked by end moraines on Long Island and Staten Island.
NASA Johnson Space Center, Imagery Services. |
| Finger Lakes, New York: NASA space-shuttle photo, STS51B-33-028,
70 mm format, 4/85. Near-vertical view of Lake Ontario and New York Finger
Lakes. Finger Lakes occupy deep, ice-carved valleys that diverge southward
from the Ontario basin. South of the Finger Lakes, a network of river valleys
marks the path of ice-marginal melt-water drainage related to the Ontario ice
lobe. NASA Johnson Space Center, Imagery Services. |
| Lake Michigan and Green Bay, WI/MI: NASA space-shuttle photo,
STS28-153-22, 5-inch format, 8/89. High-oblique view toward southwest over
Lake Michigan and Green Bay (right of lake). Large ice lobes followed both
basins toward the south; ice flow was split by bedrock high that forms
Green Bay peninsula and islands to the north. NASA Johnson Space Center,
Imagery Services. |
| Prairie Coteau, MN/SD: NASA space-shuttle photo, STS36-152-110,
5-inch format, 3/90. Near-vertical view of Coteau des Prairies, eastern
South Dakota and western Minnesota. Lakes are frozen, but ground is snow
free in this late winter scene. The prominent light-colored zone along the
eastern edge is the Bemis Moraine, which formed along the western side of
the Des Moines lobe during late Wisconsin glaciation. Small elongated lakes
in the moraine occupy tunnel valleys that were eroded by melt water flowing
out from beneath the ice lobe. NASA Johnson Space Center,
Imagery Services. |
Chamberlin undertook detailed mapping and analysis of
the glacial features associated with the Green Bay lobe. From this he developed
three fundamental laws of glacier dynamics in ice lobes (Chamberlin 1886).
- A broad depression, reaching well backward along the line of glacial
movement, was effective in producing prolongations of the ice (p. 183).
Horberg and Anderson (1956) reached a similar conclusion, noting that
bedrock topography was most important for controlling the form of ice lobes.
Broad, bedrock troughs of the Great Lakes are prime
examples of this phenomenon--a major ice lobe was active in each.
| Great Lakes, United States and Canada: NASA space-shuttle photograph, STS064-54-022,
9/94, 5-inch format. High-oblique view toward southwest with sunglint from lake surfaces;
Lake Ontario to left, Lake Michigan to far right. Great Lakes basins were eroded
by large ice lobes that spread southward from the Laurentide Ice Sheet.
NASA Johnson Space Center, Imagery Services. |
- In glacial flow as applied to the marginal portions of the ice ...
the movement was essentially at right angles to the border (p. 184).
Thus, ice movement ascended from basins toward highlands; a radial or
divergent flow pattern developed in the ice lobes.
- Ice overriding in a deep current covering all topographic features will
be less affected by these topographic features, which produce deflections
only in the basal ice flow.
| Memorial plaque to T.C. Chamberlin on a large erratic boulder, University of
Wisconsin, Madison. Chamberlin's service to Wisconsin and geology are commemorated on
this monument. Photo © by J.S. Aber. |
The various glacial landforms are usually arranged in systematic patterns
relative to ice lobes. End moraines, ice-pushed hills, and kames define arcs or crescents that outline the
margins of ice lobes. Landforms associated with melt-water erosion or
deposition in streams, lakes, or seas are located beyond the edges of ice
lobes. Subglacial landforms, such as drumlins, eskers, tunnel valleys, and
ground moraine, are found within the areas occupied by ice lobes. The
kinds of landforms created by an ice-lobe advance and retreat depend on
many factors: rate of advance, substratum lithology, basal temperature, etc.
The shape, thickness, and rate of movement of a glacier lobe are controlled
by many variables--substratum topography, ice supply from interior of ice
sheet, basal melt-water conditions, nature of substratum sediment,
permafrost, etc. Two general forms of ice lobes were developed
along the southern margin of the Laurentide ice sheet (Clark 1992).
- Relatively thick lobes with steep margins, such as the Green Bay
lobe. These lobes presumably advanced over permafrost
by a combination of plastic flow and limited basal sliding. Such lobes
were able to maintain continued movement for an extended period of time,
which resulted in well-developed drumlin fields, lacking eskers and tunnel
valleys, behind end moraines.
- Relatively thin lobes with gentle margins, such as the Lake
Michigan lobe. These lobes advanced rapidly over
thawed ground by surging on water-lubricated beds or deforming beds.
Repeated advances alternated with ice stagnation; hummocky moraine, eskers,
tunnel valleys and other geomorphic features of stagnant ice are common.
Some ice lobes, that were thick and slow during early phases of ice
advance, changed to thin and fast lobes during later stages of glaciation
(Patterson 1993).
Ice lobes are the marginal expressions of ice streams--narrow zones of high-velocity flow within the ice sheet. In general, ice streams are associated with topographic depressions or areas of soft-sediment substratum, wherein subglacial meltwater or substratum deformation aided rapid ice movement. However, Stokes and Clark (2003) have demonstrated paleoice streams also in regions with hard bedrock and without topographic control. Apparently ice streams may function as a "relief valve" to ice sheet mass balance and could be initiated by a proglacial lake that destabilized (floated) the ice margin. Several large paleoice streams have been identified in the late Pleistocene Laurentide ice sheet on the basis of elongated drumlins and large glacial lineations.
Continental glacial landscape zones
The distribution of glacial erosion, deformation, and deposition for large ice sheets is not random or haphazard, but follows certain broad patterns (Dyke and Prest 1987; Aber 1992, Aber and Ber 2007). Three zones of glacial landscape modification may be recognized for continental ice sheets.
- Outer zone: Thick and nearly continuous glacial sediment of
various kinds, large end moraines, much ice-push deformation of soft
substratum. Plains and lowlands located beyond shield/mountain areas and
underlain by sedimentary bedrock; may also be found in interior positions.
Lobate style of glaciation is the rule with multiple tills and interglacial
deposits commonly preserved.
| Thick sequence of glacial strata exposed in Golden Valley bluffs, near
Medicine Hat, Alberta. The bluffs reveal >80 m of glacial sediments that
form a blanket, which buries the preglacial landscape of southern Alberta.
Photos © by J.S. Aber. |
| Streeter Moraine in Logan County, southeastern North Dakota. This moraine
is located on the Missouri Coteau; it was formed along the western margin of
the James ice lobe. The moraine contains many large ice-shoved hills, like
the example seen on the horizon. |
| Glacial sequence exposed in quarry near Freienwalde, northeastern Germany.
The light-colored mass at top is a bedrock raft (megablock) of Oligocene
glimmersand (micaeous sandstone) that was thrust over the brown-colored
glacial sediment underneath. |
| Cape Cod, Massachusetts: NASA space-shuttle photograph, STS071-708-040, 07/06/95,
70 mm format. Low-oblique view toward northwest. Cape Cod, Nantucket, Martha's Vineyard, and
other offshore islands consist of moraines and ice-shoved hills built along the margins of
ice lobes during the late Wisconsin glaciation, around 18,000 to 20,000 years ago.
NASA Johnson Space Center, Imagery Services. |
- Intermediate zone: Thin and discontinuous glacial sediment
with large areas of exposed basement rocks. Located in shield/mountain
areas of crystalline bedrock. Long eskers and glacial lineaments, but
few end moraines and little ice-push deformation. Deposits date mainly
from final phases of the last glaciation.
| View over crystalline shield terrain, Stockholm, eastern Sweden.
The hill top is exposed bedrock without any sediment or soil cover. Lower on the hill
sides, thin moraine cover is present--see next photo. This bedrock landscape is
typical of the intermediate zone of glaciation. |
| Closeup view of thin moraine cover on lower portion of bedrock
hill. The sediment is only one boulder-layer in thickness. |
- Inner zone: Moderately thick and continuous glacial sediment
with ribbed and Rogen moraine, drumlins, and common ice-push deformation
(Aber and Lundqvist 1988). Central shield/mountain areas and/or sedimentary
substratum. Crust was depressed 100s of m; multiple tills and deposits
predating last glaciation are commonly preserved.
| Andersön, a small drumlin (background) within Storsjön lake,
central Sweden. Glacial sediment forms a near-continuous cover in foreground.
The drumlin and sediment cover are typical of the inner zone of glaciation--see
next photo. |
| Closeup view of exposure on side of Andersön drumlin.
Fine sand/silt is folded around a core of coarse gravel. Overriding ice
molded the ice-pushed hill into a small drumlin. Ice movement from right to
left; scale pole is 2 m long. |
It is clear from this zonal pattern that evidence for past glaciation would
most likely be preserved in the outer zone, especially on continental
shelves and in adjacent marine basins, where deposits 100s of m thick could
accumulate. Evidence for ancient glaciation would, conversely, consist of
a vast erosional surface over much of the interior land area.
| View over Egersund, southwestern Norway. The crystalline bedrock was stripped
of soft sediment cover during repeated ice-sheet glaciations. The erosion
surface is the only evidence for former glaciation, except for scattered
pockets of sediment that date mainly from final phases of the last
glaciation. |
Glacially scoured tundra landscape of
Magerøya, northernmost Norway.
The Antarctic shelf shows the unusual effects of repeated glaciations.
Most continental shelves are <200 m deep near land and become gradually
deeper (1 km) toward the shelf margin. The Antarctic shelf is the
opposite. The shelf is relatively deep (0.8-1.3 km) near shore, but becomes shallower toward the margin, where water depth is
only 200-400 m (ten Brink and Schneider 1995). Greater depth of the inner
shelf results from glacial erosion and/or non-deposition; whereas the outer
shelf has built up a very thick sequence of glacially related deposits.
The Greenland continental shelf exhibits similar development, in which
outer portions have end moraines preserved in quite shallow water, and deep
channels are cut across inner portions.
These landscape zones are related in general to the availability of
erodible or deformable substrata, namely thick sediments or sedimentary
bedrock. The three glacial landscape zones are the results of multiple
glaciations and represent long-term, cumulative modifications of
continental substratum by ice sheets. Complex patterns of ice movement
exist, particularly in the inner and intermediate zones of glaciation, due
to changing ice centers and flow lines. Thus, the landforms of older glacial movements may be overprinted, much modified, or
completely obliterated by later ice flows. The results are cross-cutting geomorphic patterns over regions of former glaciation.
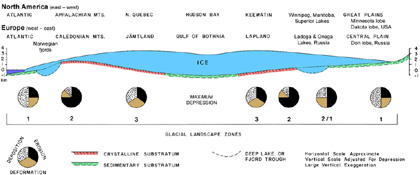
Model of glacial landscape symmetry for North America (east-west) and Europe (west-east) with schematic ice-sheet profile, substratum bedrock, and crustal depression. Pie diagrams represent local, relative contributions of glacial erosion, deformation, and deposition for modifying the three landscape zones (1-3); overall magnitude of glacial landscape modification increases outward to the east and west. Adapted from Aber (2022).
| |
The three glacial landscape zones are apparently also related to thermal
regimes developed at the base of the ice sheets, particularly during
deglaciation. The glacial landscape zones in North America and northern Europe are arranged in symmetrical patterns with
respect to the Atlantic Ocean. The general correspondence in glacial landscapes is most remarkable considering the
geographic and climatic differences between the two continents. Repeated
ice-sheet glaciations have imposed a common geomorphic pattern, in spite of
these differences.
Glacial landscape patterns on Mars
Recent claims of evidence for past life on Mars have reignited popular and scientific
fasination with the red planet, even though modern Mars is clearly unsuitable for life
as we know it on the planet's surface. Mars has a CO2-rich atmosphere, but the
atmosphere is so thin (0.006 bar) that it has little greenhouse effect. Mars has a
water-rich crust, in which a huge volume of water is locked in permafrost. Frozen
CO2 (dry ice) and water ice form the seasonal polar ice caps. Mars was once
warmer and may have had as much as 1-5 bars CO2 pressure in its atmosphere.
Landforms of the martian surface provide abundant evidence for the past existence of liquid
water, oceans, sea ice, and even glaciers (Kargel and Strom 1996).
The infamous canals of Mars are really giant valleys eroded by immense water
floods. These floods were triggered when episodic volcanism melted large
volumes of permafrost in the Tharsis Bulge region.
The floods scoured channel networks leading to a large basin in the
northern hemisphere, in which Oceanus Borealis was formed. Oceanus
Borealis covered up to 40 million km² with an average water depth of about
1700 m (1 mile). Such oceans may have formed repeatedly following major
volcanic outbursts.
During each oceanic phase, a mild climate with moderate greenhouse effect
came into being. Water vapor was circulated in the atmosphere around the
planet. Snowfall and glacier formation occurred in the southern hemisphere
resulting in a vast Austral Ice Sheet. The array of glacial
landforms present on Mars bears a striking similarity to glacial features
of the Earth. The mild climatic episodes on Mars evidently
were short-lived, however, because volcanism was not continuous. The
climatic history of Mars apparently consisted of long, cold intervals of
permafrost punctuated by short, mild, oceanic/glacial episodes. The final glacial epoch took place late in martian history, not less than about 100,000 years nor greater than 20 million years ago (Kargel and Strom 1992).
The ultimate failure of Mars' episodic greenhouse climate can be
attributed to the planet's small size. The planet's interior cooled early
in its history, and volcanic emission of CO2 became intermittent. Weak
gravity allowed much of the atmosphere to escape, so that a permanent
greenhouse effect and mild climate could not become established.
This volcanic-climatic scenario along with the chance of martian life remain highly controversial subjects among planetary scientists and the public. The possibility of past life on Mars has major theological as well as scientific implications. A series of NASA missions are designed to collect data from martian atmospheric and surficial environments. These unmanned missions may culminate ultimately in manned expeditions by the mid-21st century. Only then could we firmly establish the evidence for past glaciation and the possibility of ancient life on Mars.
Related sites
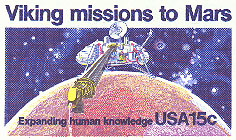
Glossary or references.