Remote Sensing Basics
Remote sensing is the observation and measurement of objects from a
distance, i.e. instruments or recorders are not in direct contact with
objects under investigation. Remote sensing depends upon measuring
some kind of energy that is emitted, transmitted, or reflected from an
object in order to determine certain physical properties of the object. One
of the most common types of remote sensing is photography, which along with
many other techniques is utilized for the images in this Lewis and Clark atlas.
These techniques are based on sensing electromagnetic energy emitted or reflected from the Earth's
surface and detected at some altitude above the ground. The electromagnetic spectrum is, thus,
the starting point for understanding remote sensing. Passive remote sensing is based
on detecting available (background) electromagnetic energy from natural sources, such as sunlight.
Active remote sensing, in contrast, depends on an artificial "light" source, such as radar,
to illuminate the scene.
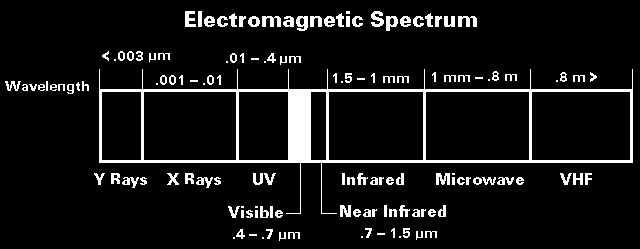
Taken from U.S Geological Survey EROS Data Center--see EDC.
Sunlight is the main source of energy at the Earth's surface with most
energy in the ultraviolet, visible, and short infrared portions of the
spectrum. The Earth is a much weaker source of energy at
longer wavelengths of thermal infrared and microwaves. All passive
remote sensing is based on these two energy sources.
- Ultraviolet, visible, and near-infrared radiations (< 3 µm wavelength) are mainly
reflected solar energy.
- Mid-infrared, thermal-infrared, and microwave radiations (> 3 µm wavelength) are mostly emitted
from the Earth's surface.
Aerial Photography
Photography is one of the oldest and most versatile forms of
remote sensing of the Earth's surface. All photographic cameras have
certain basic components: lens, diaphragm, shutter, viewfinder, and
image plane. Geometry of the lens and film format determine the
scene area focused onto the image plane. The diaphragm and shutter
control the amount of light to expose each photograph.
The spectral sensitivity of photography ranges from about 0.3 µm (near-ultraviolet)
to 0.9 µm (near infrared).
Different parts of the spectrum may be photographed by using
various combinations of films and filters. Photographs are routinely taken in b/w
panchromatic, b/w infrared, color-visible, color-infrared, and multiband types.
For example, color-infrared film is exposed to green, red, and near-infrared wavelengths,
which are depicted as blue, green and red in the photograph. This shifting of bands to
visible colors is called false-color.
Manned-Space Photography
U.S. manned space photographs of the Earth began with the Mercury,
Gemini, and Apollo missions of the 1960s. Many spectacular
images of the lands, oceans, and atmosphere were obtained, and
these demonstrated the potential of synoptic space photography
for various earth science investigations. With few exceptions,
these photos were taken at the discretion of astronauts, who had
little formal background in either photography or earth science.
Systematic manned space photography was undertaken during
the Skylab missions of 1973 and 1974. Skylab was a orbiting
space station that was utilized for extended Earth observations.
Skylab 4 was most successful; about 2000 photographs were
obtained of more than 850 features and phenomena (Wilmarth et al.
1977). The lessons learned during Skylab missions formed the basis for
the program of space-shuttle photography in the 1980s and '90s.
| South Island of New Zealand.
|
| Chicago and Lake Michigan, Illinois. |
Astronauts on space-shuttle missions have taken more than
250,000 photographs with hand-held cameras. A large portion of
these are Earth-looking images that provide unique views of the
world's surface features: deserts, volcanoes, mountains, coasts,
oceans, glaciers, sea ice, rivers, lakes, dust, fires, clouds,
and human settlement. Space-shuttle photographs are taken in all
possible orientations--near vertical, low oblique, and high
oblique--for a greater range of perspectives than is possible
with any unmanned satellite instruments.
Space-shuttle photography is the result of systematic Earth
and environmental science training given to each astronaut crew
prior to flight. Much of the photography consists of revisits to
targets previously photographed. Each day during flight,
astronauts are given instructions for photographs based on cloud
cover and orbital conditions. Astronauts are also free to take
photographs of any interesting or attractive scenes. Special
advantages of astronaut photography include the following (Lulla et al. 1993).
- Images are taken at various sun angles, ranging from negative
angles (below horizon) to nearly vertical. This
provides for unique views under various lighting conditions
that are not available with other remote sensing systems.
- Sequential (overlapping) near-vertical photographs with
different look angles provide for stereoscopic viewing.
- Little-known tropical areas are well represented in the
photography database. These regions are undergoing very
rapid human-induced environmental transformations.
Various cameras, lenses, and film types are employed for
space-shuttle photography. Hasselblad cameras are most commonly
used. These take pictures with 70-mm (2¾-inch) format film.
Lenses vary from 40 mm to 250 mm focal length. The Linhof camera
is also utilized on most missions; it takes 125-mm (5-inch) format
film. Other still cameras that have been used include Rolleiflex
(70 mm) and Nikon (35 mm). IMAX and 16-mm movie footage are taken
on some flights along with video imagery. The electronic still camera
(ESC) is a charged-couple device that produces near-film-quality images
in digital format. It has been utilized on several missions since 1991.
Current spectral response range is 0.4 to 1.1 µm, which is greater
than films now in use (Lulla and Holland 1993).
Film selection for space-shuttle photography is relatively
conservative. Most photographs are exposed on color-visible
films of various types. Color-infrared film is used occasionally,
and other special film types are employed rarely. Most
photographs have been digitized and converted to video format.
"Digitization, rectification, multi-layering (GIS), classification,
and mensuration of these digitized analog images is now
fairly routine ..." (Lulla et al. 1993).
| Florida, Gulf of Mexico, and Atlantic Ocean.
|
| Smoke from forest fires in Queensland, Australia. |
| Color-infrared view of Bangkok, Thailand.
|
| Color-infrared view, Mt. St. Helens, Washington. |
Time of launch and crew sleeping schedules affect the times
of daylight when photographs may be acquired, as very few pictures
are taken on the Earth's night side. Thus, some missions
return pictures mainly from the northern hemisphere, whereas
other missions take photos mostly in the southern hemisphere. An
important factor is cloud cover that often obscures ground features
of interest. For example, certain equatorial sites have
little or no cloud-free photographs. Photography is not the
primary objective on most space-shuttle flights, so pictures are
taken when astronauts are not busy with other duties. The result
of these factors is very uneven coverage of the world.
The presence of human photographers in space provides some
important capabilities: to observe and respond quickly to unusual
ground events, to preselect and acquire scientifically useful
images, and to photograph phenomena from different look angles.
"These qualities demonstrate the value of a human observer in
orbit in adding to understanding of our planet Earth, and
interacting with ground scientists in real time to acquire data
and confirm or deny events." (Lulla and Helfert 1991)
More information
NASA Johnson Space Center--Imagery services.
NASA Johnson Space Center--Earth from space.
| Natural-color composite. Image consists of blue,
green, and red visible light portrayed in a natural manner. Active vegetation appears
green, bare soil and fallow fields are brown, and urban structures are white. Clean water
bodies appear black, whereas the Missouri River displays a muddy brown color. Landsat TM bands
1, 2, 3; image processing by J.S. Aber ©. |
| Standard false-color composite. Image consists of green,
red, and near-infrared light portrayed in a false-color manner. Active vegetation appears
red-pink, bare soil and fallow fields are green, and urban structures are bluish-white. Clean
water bodies appear black, whereas the Missouri River displays a green-brown color. Landsat TM
bands 2, 3, 4; image processing by J.S. Aber ©. |
| Special-color composite. Image consists of red,
near-infrared and mid-infrared light portrayed in a false-color manner. Active vegetation
appears green, bare soil and fallow fields are red-brown, and urban structures are purple.
Most water bodies are black, and the Missouri River appears dark blue. Landsat TM bands 3,
4, 5; image processing by J.S. Aber ©. |
| Special-color composite. Image consists of green,
near-infrared and mid-infrared light portrayed in a false-color manner. Active vegetation
appears green, bare soil and fallow fields are red-brown, and urban structures are purple.
Most water bodies are black, and the Missouri River appears dark blue. Landsat TM bands 2,
4, 7; image processing by J.S. Aber ©. |
| Vegetation image based on red and near-infrared bands.
Active vegetation is green; dormant or dry plants are yellow; plowed fields, pavement, and
bare ground are brown. All water bodies appear black. Landsat TM bands 3 and 4 utilized to
create the normalized difference vegetation index--see
NDVI.
Image processing by J.S. Aber ©. |